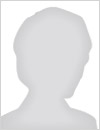
METIS is the European Extremely Large Telescope (ELT) 1st-generation Mid-Infrared ELT Imager and Spectrograph. It will offer spectroscopic, imaging and coronagraphic capabilities from 3 up to 13 microns with Adaptive-Optics correction.
With its Final Design Review due late 2022 we report on the wavefront control strategy devised to meet the METIS science and technological requirements. Such strategy addresses challenging aspects as i) the appearance of differential petal piston modes in the presence of secondary mirror support struts caused either by numerical processing or the actual, physical low-wind effect, ii) the numerical pupil derotation and mis-reg compensation, iii) the adaptation to transient disturbance signals such as telescope-to-instrument handover control and iv) the compliance with constrained modal control of the pre-focal beam corrector mirrors (M4/M5).
The overall METIS wavefront control strategy consists in a split approach cemented in a sequence of steps: 1) Tikhonov-regularised spatial wavefront estimation/reconstruction on a zonal Cartesian coordinate system tied to the pyramid (P-WFS) sampling pixel grid, 2) the regularised projection onto a global modal control space including correction of mis-registrations and rotation between the P-WFS coordinate grid and the ELTs M4/M5, and 3) the time-filtering through the application of proportional-integral control before converting to actuator commands readied for the ELTs collaborative TT off-loading scheme whilst avoiding hitting the mirrors constraints in amplitude, speed and force.
We present physical-optics simulation results of the whole AO system obtained with prototyped instances of the real-time and soft-real-time computers including sensitivity analysis with respect to observational, atmospheric, non-atmospheric (telescope-intrinsic such as wind-induced low-order modes comprising tip-tilt) and instrument-specific conditions and disturbances.
An error budget is put together that meets the METIS science requirements in terms of wavefront error with reassuring margins thus endorsing the strategy devised.HARMONI is the first light visible and near-IR integral field spectrograph for the ELT. It covers a large spectral range from 450nm to 2450nm with resolving powers from 3500 to 18000 and spatial sampling from 60mas to 4mas. It can operate in two Adaptive Optics (AO) modes - SCAO (including a High Contrast capability) and LTAO - or with NOAO. The project is preparing for Final Design Reviews.
The SCAO system for HARMONI is based on a pyramid wavefront sensor (PWFS) operating in the visible (700 – 1000 nm). Previous implementations on very large telescopes have demonstrated the challenges associated with optimising PWFS performance on-sky, particularly when operated at visible wavelengths. ELT operation will pose further challenges for AO systems, particularly related to the segmentation of the telescope and the control of badly seen ‘petal modes’. In this paper we investigate these challenges in the context of the HARMONI SCAO system. We present the results of end-to-end simulations of our baseline approach, using a coupled control basis to avoid the runaway development of petal modes in the control loop. The impact of key parameters are investigated and methods for optical gain compensation and optimisation of the control basis are presented. We discuss recent updates to the control algorithms and demonstrate the possibility of improving performance using a form of super resolution. Finally, we report on the expected performance across a range of conditions.The infrared low order sensor (IRLOS) upgrade project was recently launched to increase the sky coverage of GALACSI narrow-field mode (NFM).1, 2 While the baseline is to perform low-order wavefront sensing with a 2x2 Shack-Hartmann wavefront sensor (SHWFS) operating in the J+H band, a full-pupil mode was proposed to address the faintest end of the magnitude range by concentrating the photons from the full aperture in a single point spread function (PSF). In this context, we have investigated the wavefront sensing approach called the linearized focal-plane technique (LIFT). It enables the retrieval of low-order modes such as tip/tilt, defocus, astigmatism (and possibly more) from a single focal-plane PSF of a very faint natural guide star (NGS) target. LIFT is a phase diversity technique based on introducing a known amount of astigmatism into the optical path. The morphological change induced by the astigmatic shift allows encoding information about the phase aberrations into the PSF morphology.
In this work, we discuss the linearity and flux sensitivity of the method and present experimental on-sky results obtained at the VLT. We discuss the applicability of this method in realistic conditions and the limitations that this method can face while operated on-sky.Wavefront sensors (WFSs) encode phase information of an incoming wavefront into an intensity pattern that can be measured on a camera. Several kinds of WFSs are used in astronomical adaptive optics. Among them, Fourier-based WFSs perform a filtering operation on the wavefront in the focal plane. The most well-known example of a WFS of this kind is the Zernike WFS. The pyramid WFS also belongs to this class. Based on this same principle, WFSs can be proposed, such as the
We analyze some restrictions found in the estimation process, which are general to any measurement technique. The insensitivity of the instrument to large values of outer scale is one of them, as the telescope becomes blind to outer scales larger than its diameter. Another problem is the contradiction between the length of data and the stationarity assumption of the turbulence (turbulence parameters may change during the data acquisition time).
Our method effectively deals with problems such as noise estimation, asymmetric correlation functions and wavefront propagation effects. It is shown that the latter cannot be neglected in high resolution AO systems or strong turbulence at high altitudes. The method is applied to the Gemini South MCAO system (GeMS) that comprises five wavefront sensors and two DMs. Statistical values of L0(h) at Cerro Pachón from data acquired with GeMS during three years are shown, where some interesting resemblance to other independent results in the literature are shown.
We show, in addition to the expected PSF degradation with the field direction, that the PSF retains a coherent core even at large off-axis distances. We demonstrated the large performance improvement of fine tuning the sampling frequency for dimer natural guide stars and an improvement of approx. 50% in SR can be reached above the nominal case. We show that using a smaller AO system with only 20x20 sub-apertures it is possible to further increase performance and maintain equivalent performance even for large off-axis angles.
Tomography requires the knowledge of the statistical turbulence parameters, commonly recovered from the system telemetry using a dedicated profiling technique. For demonstration purposes with the MOAO pathfinder CANARY, this identification is performed thanks to the Learn & Apply (L&A) algorithm, that consists in model-fitting the covariance matrix of WFS measurements dependant on relevant parameters: Cn2(h) profile, outer scale profile and system mis-registration.
We explore an upgrade of this algorithm, the Learn 3 Steps (L3S) approach, that allows one to dissociate the identification of the altitude layers from the ground in order to mitigate the lack of convergence of the required empirical covariance matrices therefore reducing the required length of data time-series for reaching a given accuracy. For nominal observation conditions, the L3S can reach the same level of tomographic error in using five times less data frames than the L&A approach.
The L3S technique has been applied over a large amount of CANARY data to characterize the turbulence above the William Herschel Telescope (WHT). These data have been acquired the 13th, 15th, 16th, 17th and 18th September 2013 and we find 0.67"/8.9m/3.07m.s−1 of total seeing/outer scale/wind-speed, with 0.552"/9.2m/2.89m.s−1 below 1.5 km and 0.263"/10.3m/5.22m.s−1 between 1.5 and 20 km. We have also determined the high altitude layers above 20 km, missed by the tomographic reconstruction on CANARY , have a median seeing of 0.187" and have occurred 16% of observation time.
We tour the processing of both the isoplanatic and anisoplanatic tilt modes using the spatio-angular approach whereby the wavefront is estimated directly in the pupil plane avoiding a cumbersome explicit layered estimation on the 35-layer profiles we're currently using.
Taking the case of Harmoni, we cover the choice of wave-front sensors, the number and field location of guide-stars, the optimised algorithms to beat down angular anisoplanatism and the performance obtained with different temporal controllers under split high-order/low-order tomography or joint tomography. We consider both atmospheric and far greater telescope wind buffeting disturbances. In addition we provide the sky-coverage estimates thus obtained.
The Pyramid now forms part of the baseline for several next generation Extremely Large Telescopes (ELTs). As such its behaviour under realistic operating conditions must be further understood in order to optimise performance. At LAM a detailed investigation into the performance of the Pyramid aims to fully characterise the behaviour of this wave-front sensor in terms of linearity, sensitivity and operation. We have implemented a Pyramid sensor using a high speed OCAM2 camera (with close to 0 readout noise and a frame rate of 1.5kHz) in order to study the performance of the Pyramid within a full closed loop adaptive optics system. This investigation involves tests on all fronts, from theoretical models and numerical simulations to experimental tests under controlled laboratory conditions, with an aim to fully understand the Pyramid sensor in both modulated and non-modulated configurations. We include results demonstrating the linearity of the Pyramid signals, compare measured interaction matrices with those derived in simulation and evaluate the performance in closed loop operation. The final goal is to provide an on sky comparison between the Pyramid and a Shack-Hartmann wave-front sensor, at Observatoire de la Côte d'Azur (ONERA-ODISSEE bench). Here we present the adaptive optics setup at LAM and latest experimental and modelling results. The loop is closed on different static wave-front errors: the initial shape of the deformable mirror (DM) and a turbulent-like shape projected onto the DM. The results demonstrate a Pyramid closed loop performance of 7–8nm rms wave-front error compared to a reference at surface.
We have developed a Point Spread Function (PSF)-Reconstruction algorithm dedicated to MOAO systems using system telemetry to estimate the PSF potentially anywhere in the observed field, a prerequisite to deconvolve AO-corrected science observations in Integral Field Spectroscopy (IFS). Additionally the ability to accurately reconstruct the PSF is the materialization of the broad and fine-detailed understanding of the residual error contributors, both atmospheric and opto-mechanical.
In this paper we compare the classical PSF-r approach from Véran (1) that we take as reference on-axis using the truth-sensor telemetry to one tailored to atmospheric tomography by handling the off-axis data only.
We've post-processed over 450 on-sky CANARY data sets with which we observe 92% and 88% of correlation on respectively the reconstructed Strehl Ratio (SR)/Full Width at Half Maximum (FWHM) compared to the sky values. The reference method achieves 95% and 92.5% exploiting directly the measurements of the residual phase from the Canary Truth Sensor (TS).
View contact details